Koch-Haaf Carbonylation
In this tutorial, I want to talk about an industrially important method for synthesizing carboxylic acids, known as the Koch-Haaf carbonylation reaction. Typically, this involves reacting an alcohol with carbon monoxide in the presence of a strong acid like sulfuric acid. The result is the formation of a carboxylic acid.
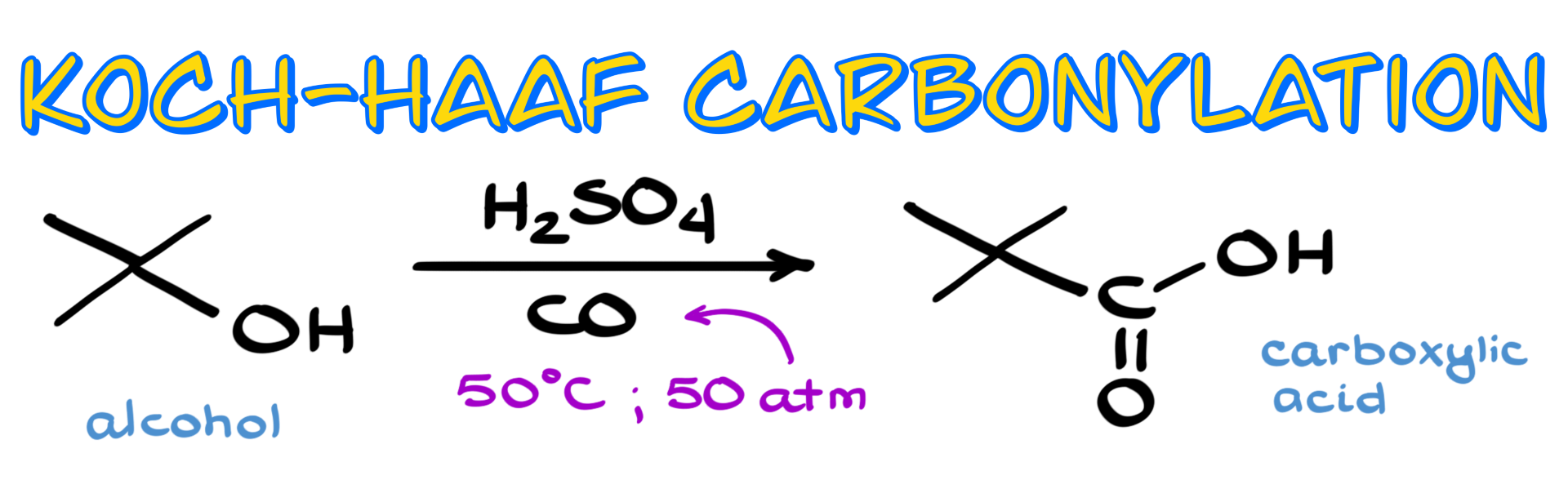
The reaction usually takes place under relatively mild temperatures—around 50 °C. However, the pressure of the carbon monoxide is a different story. It generally requires about 50 atmospheres, which is quite high and definitely not something you’d casually run in a standard lab setting.
Mechanism of the Koch-Haaf Carbonylation
Now, let’s get into the mechanism.
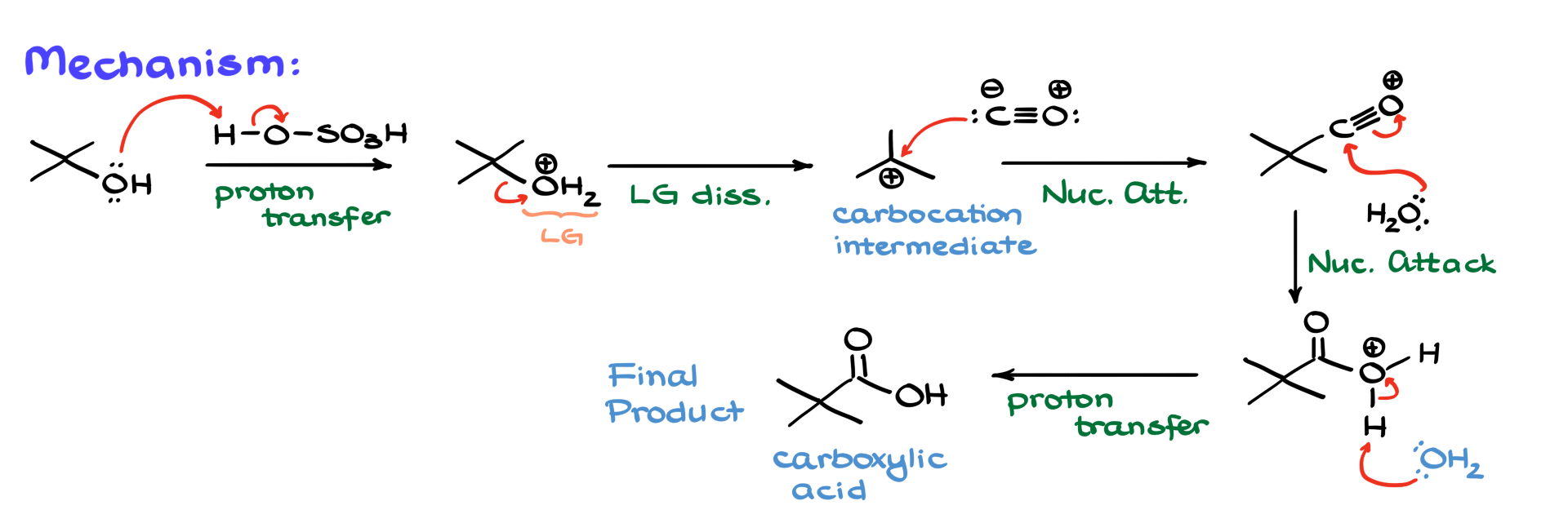
We begin by protonating our alcohol using sulfuric acid, which gives us an intermediate where we’ve converted the -OH group into H₂O—a fantastic leaving group. This water molecule then leaves, generating a carbocation intermediate. Since the reaction proceeds through a carbocation—primary alcohols typically don’t work well, and even secondary ones can be problematic. If you do use primary or secondary substrates, you’ll likely encounter carbocation rearrangements.
Next, carbon monoxide steps in. I’m showing the Lewis structure here to highlight that the carbon in CO carries a partial negative charge, making it nucleophilic. So, that carbon attacks the carbocation, forming a new carbon-carbon bond and giving us an acylium ion as the intermediate. What I’m showing here is the dominant resonance structure, but you can also draw the one with the positive charge on carbon.
From this point, water that is typically present in the reaction acts as a nucleophile and attacks the acylium ion, forming a bond between carbon and oxygen. Then, all that’s left is a proton transfer. Another water molecule or any base-like species grabs the extra proton, and just like that, we have our carboxylic acid.
Koch-Haaf Carbonylation Variations
One of the really cool things about this reaction is that it doesn’t matter how you generate your carbocation.
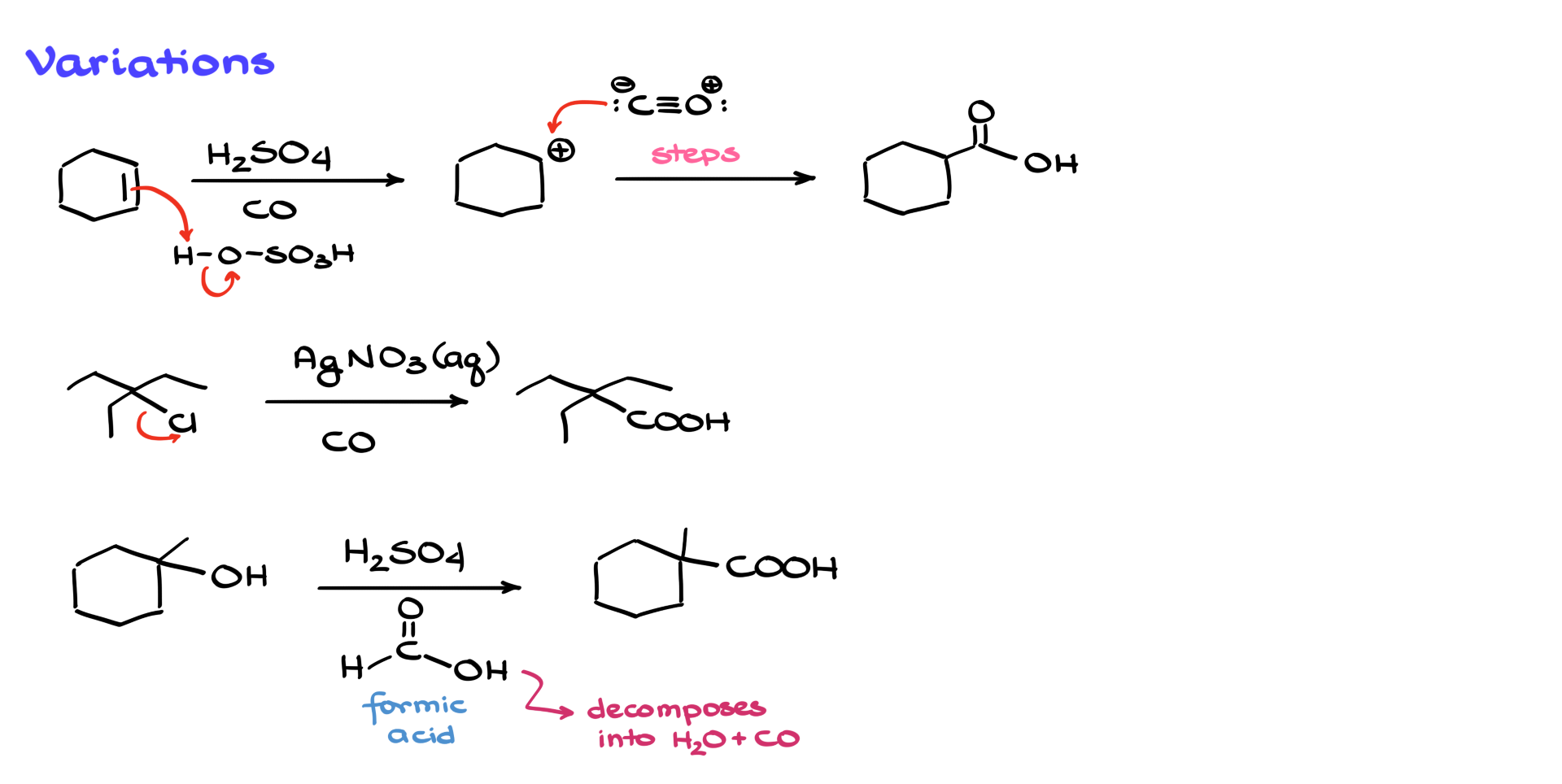
For example, let’s say we start with cyclohexene. If we react it with sulfuric acid, the π bond attacks a proton from the acid and that gives us a carbocation. Once we’ve got the carbocation, everything else proceeds just like before: carbon monoxide attacks, forms the acylium ion, water attacks, proton transfer happens, and you get the carboxylic acid. So even though we started with an alkene, the core mechanism doesn’t change once the carbocation is formed.
Alternatively, we can use alkyl halides. If we have an alkyl halide, we can remove the halogen using strong Lewis acids like iron(III) chloride or aluminum chloride. Another popular method is using silver(I) ions (Ag⁺) will react with the halide, forming insoluble silver chloride and generating the carbocation in the process. Once that carbocation forms, the mechanism continues as before and we end up with the corresponding carboxylic acid.
Mechanistically, it’s always the same story: you start with a leaving group dissociation to form a carbocation, then carbon monoxide attacks, followed by water, and a final proton transfer gives you the acid. The downside to this method (and the main reason it’s not often used in labs) is the need for high-pressure carbon monoxide. And let’s not forget, carbon monoxide is extremely toxic, so handling it at high pressure is dangerous and definitely not something we want to mess with in a small lab.
So, what’s the alternative? Well, instead of using gaseous CO directly, we can generate it in situ using formic acid. When you mix formic acid with a strong mineral acid like sulfuric acid, the formic acid decomposes into carbon monoxide and water. This way, you’re producing CO right in the reaction mixture, under much milder conditions. Sometimes, you can even run the reaction at room temperature. You avoid using high-pressure CO, and still get your carboxylic acid.
The catch? Carbocation formation. As you probably know, carbocations come with a whole set of problems—chief among them being rearrangements. I didn’t show any rearrangement examples in this tutorial, but as soon as you try to form a carbocation on a primary or secondary carbon, you’re just asking for trouble. Even tertiary carbocations can rearrange. That’s why the yields from this reaction aren’t always great and why it’s mostly reserved for industrial use. In a lab, we care about yields and avoiding messy side products, so a method prone to carbocation rearrangements isn’t exactly welcome. But in industry, where reactions are scaled up and a bit of inefficiency can be tolerated, it’s a different story.
So, what do you think about this reaction? Have you ever seen it before? Let me know in the comments.