Directing Effects in Electrophilic Aromatic Substitution Reactions
In this tutorial, I want to talk about the directing effects in electrophilic aromatic substitution reactions. So, let’s start by looking at this reaction.
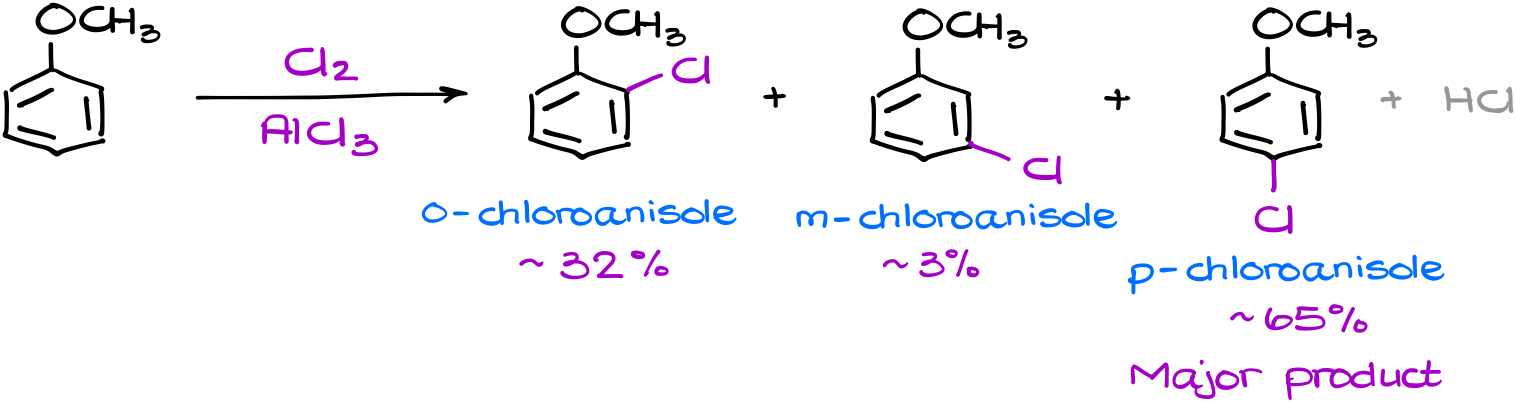
Since this is no longer a simple benzene molecule, we can make three different products. And of course, when I say three products, I only mean the organic compounds as we don’t really care about the HCl here, which is our co-product. We’ll call them the ortho-, the meta-, and the para-products. And if we were to ignore any electronic effects, we would expect a roughly equal quantities of these products in the resulting mixture. However, the reality is always a bit more interesting than our expectations.
In reality we have a clear major product, a minor one, and only trace amounts of the last one. And what is even more interesting is that the major product is the one that we would probably not even consider as one from the pure statistical perspective. So, what’s going on here? How can we explain this observation?
Resonance in Substituted Benzenes
First, let’s look at the resonance structures in the anisole itself.
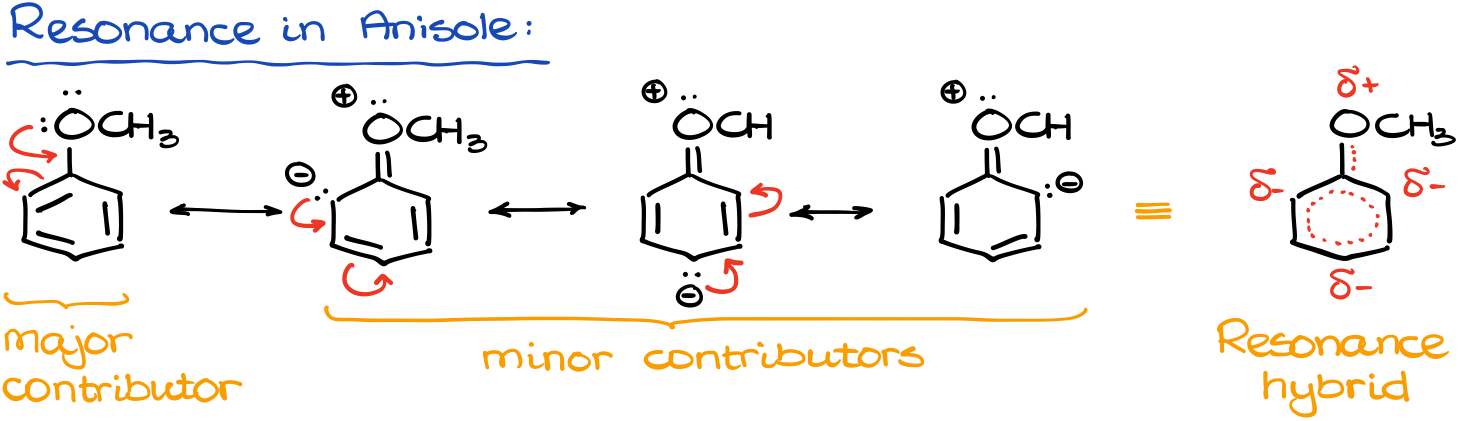
We have the uncharged major contributor, and three charged minor contributors. But what is more important, it’s the structure we get when we combine those all into an overall hybrid. By looking at the hybrid, we get some idea of the electron density distribution in our molecule. We can see that we have a higher electron density on the ortho- and -para-atoms of the aromatic ring. We show that with the δ- symbol. And as those positions in our molecule have an excess of electron density, they are going to be more reactive in reactions with electrophiles. In other words, electrophiles, which are electron-deficient, will be more attracted to those positions.
Since the methoxy group (-OCH3) pushes the electron density into the ring making it more reactive with electrophiles, we are going to call groups like that the “activating” groups.
Let’s continue our investigation of this reaction and look at the mechanism in more details.

The first step is the electrophile formation.
Next, our electrophile will react with our anisole. And this is where the interesting part is. At this point we can attack our molecule at three different positions. This will give us three different carbocation intermediates (σ-complex).
So, since we have three different resonance-stabilized intermediates, we need to draw all resonance structures for them to see if there’s any difference.
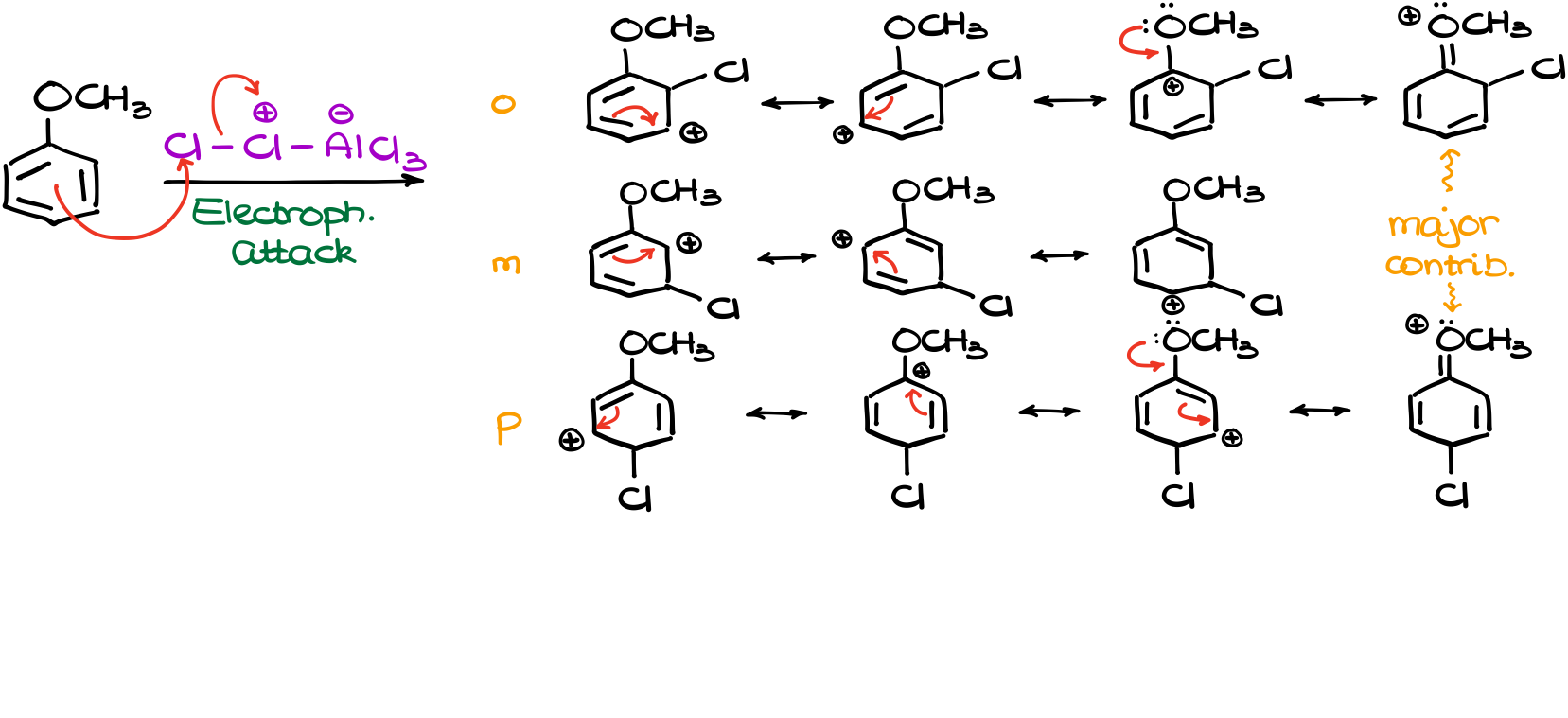
And once I have all my resonance structures together, I can see that in the case of the o- and p-intermediates, we have four resonance contributors while the m-intermediate only shows three. On top of that, the o- and p-carbocations have the major contributor. This means that those two σ-complexes will be more stable and will form faster. Remember, the more stable intermediates are typically favored in reactions. And since they have a comparatively lower activation energy leading to their formation, they form faster.
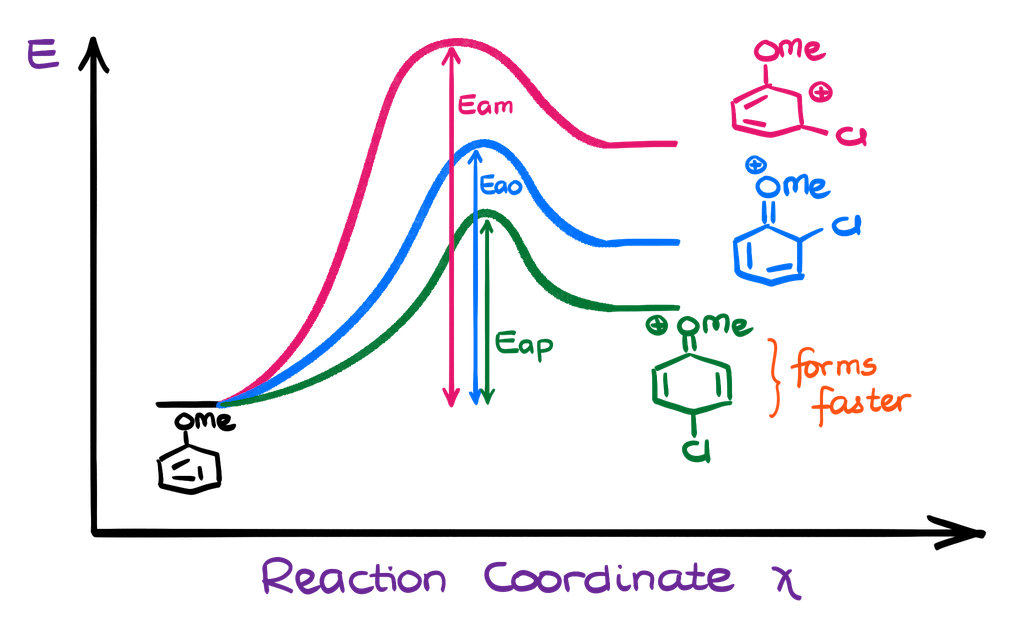
We can also represent it as the energy diagram. This energy diagram is not to scale. Nonetheless, we can see that since the activation energy of the para-intermediate (Eap) is the lowest, this intermediate will form faster than the other ones. The ortho-intermediate is a little less favorable mostly due to the steric hindrances from the methoxy group (-OCH3). There are also some electronic effects that would make ortho intermediate formation less favorable. But let’s not overcomplicate it for now.
So, going back to our mechanism, since the para-intermediate forms faster than the other ones, we would expect that to yield the major product in this reaction. Thus, if you need to explain this regioselectivity on the test, you can give the two-fold explanation. First, we have the resonance in the anisole molecule itself making the o/p positions more attractive for the electrophile. And second, the o/p resonance-stabilized intermediates (σ-complex) is more stable due to the resonance participation from the oxygen atom.
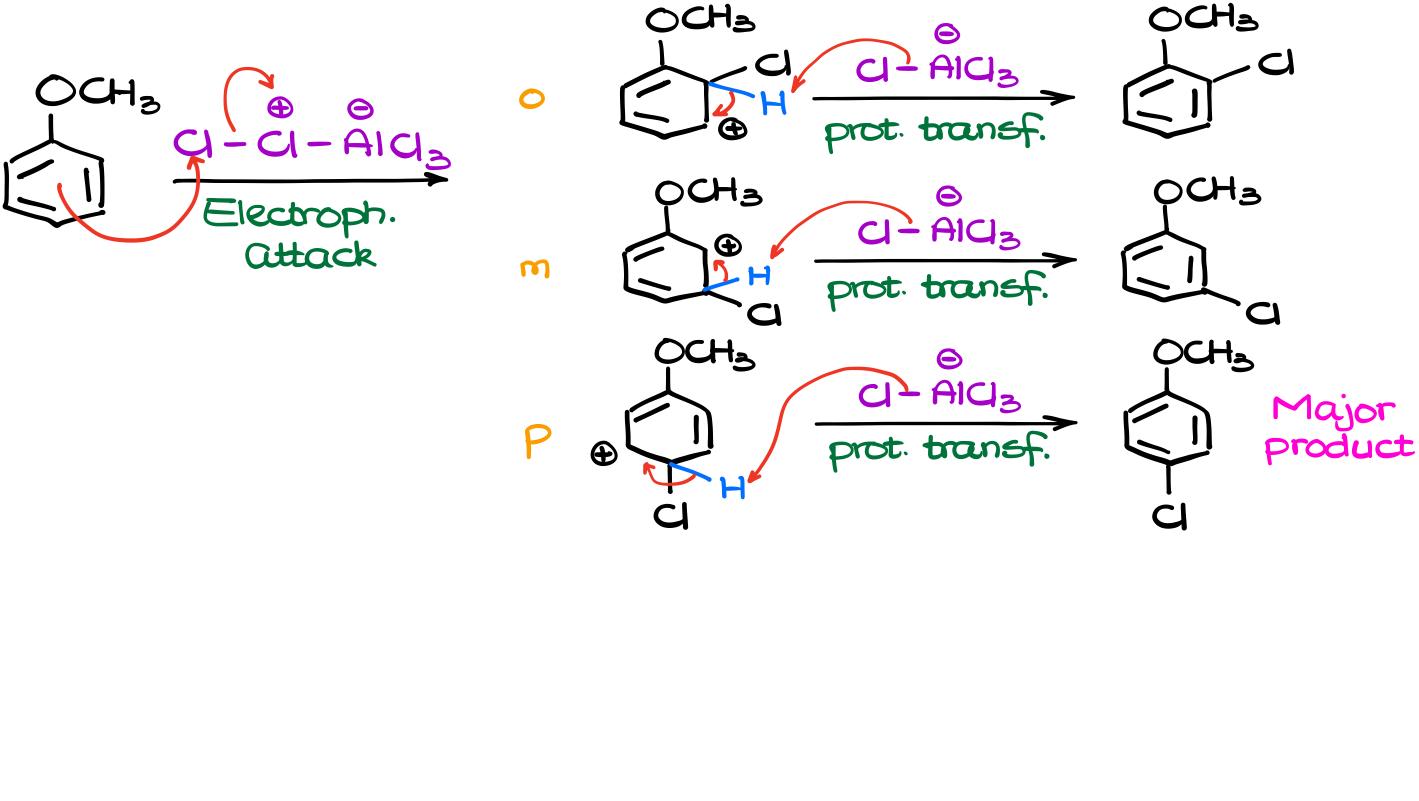
As I’ve mentioned earlier, we call groups like the methoxide group (-OCH3) in anisole the “activating” group because it makes the molecule more attractive for the electrophile. And since they direct the substitution in the o/p-positions, we also call them the ortho/para directors. Also, in most cases, the p-product will be favored over the o-product. It’s not 100% of time, but often enough so we consider that as sort of a “soft rule” for these reactions.
Activating Groups
Now, are there any other activating groups? Well, of course there are!
We’re going to distinguish between the strongly activating groups, moderately activating groups, and weakly activating groups.
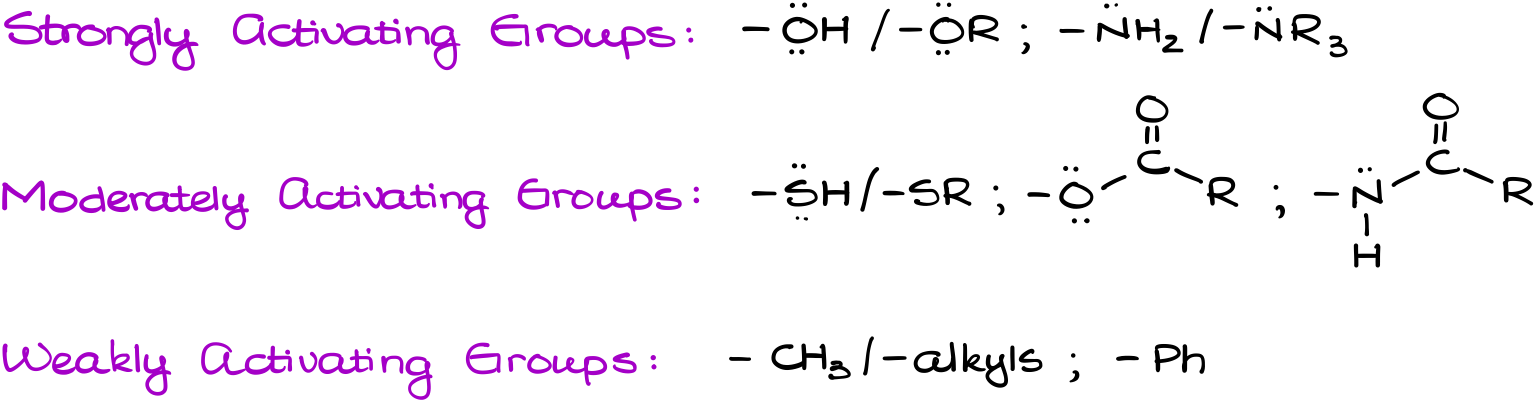
Strongly activating groups will both activate the ring and stabilize the σ-complex via the resonance. Moderately activating groups still have a resonance effect on both the ring and the σ-complex, however, their effect is much smaller. The weakly activating groups though, have negligible effect on the ring and will only help stabilize the σ-complex. So, the weakly activating groups will typically show the worst regioselectivity. They will also make the aromatic compound only marginally more reactive towards the electrophiles.
Also, all activating groups are the o/p-directors.
Deactivating Groups
Well, if there are activating groups, there must be also deactivating groups, right? And yes, there are! Let’s look at this example.
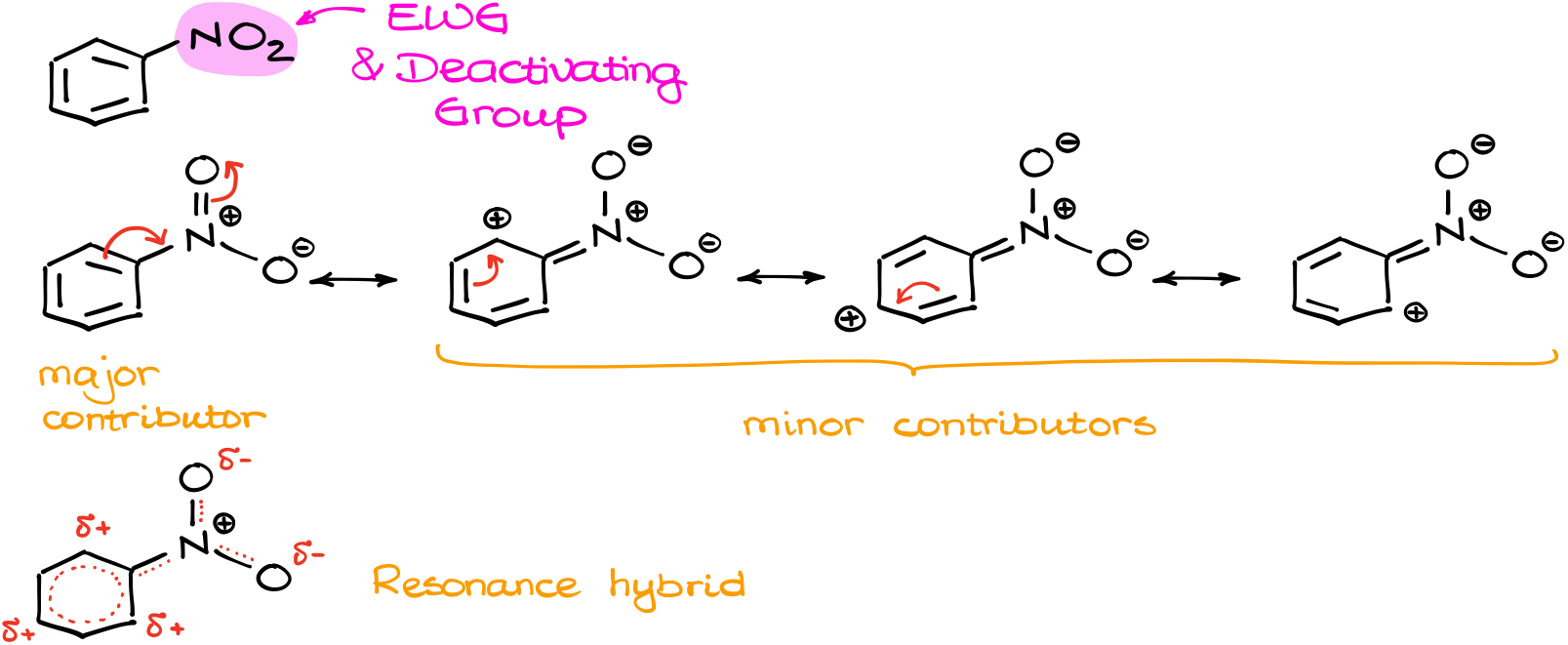
The nitro group is a typical Electron Withdrawing Group (EWG) and because of that, it is a deactivating group.
Let’s do the similar analysis we did for the methoxy group (-OCH3) in anisole. First, let’s draw the resonance structures for the nitrobenzene and see how the electron density is distributed throughout the molecule.
I’ll start by showing the full structure of the nitro group. This bit is important to see how it’s going to pull the electrons out of the ring. In resonance, we typically move the electron density towards the plus or towards the electronegative element. If we do this here, we’re going to get the following resonance contributors (figure above).
Of course, like in the case with anisole, the major contributor here is going to be the one with the least number of charges and complete octets. All minor contributors here give us open shells, which is rather unfavorable for the overall stability.
But what it does tell us, is that in the overall hybrid we’ll see the electron-depleted aromatic ring with lower electron density in the ortho- and para-positions.
This means two things:
- First, the molecule is going to be less reactive towards the electrophiles.
- Second, the o/p-positions in this molecule are deactivated because they are partially positive. Thus, even if the reaction with an electrophile were to happen, it will most likely go into the meta-position.
For instance, if we were to do this reaction, we’d see the major product being the meta-chlorinated molecule and the o/p-isomers would be present in a very negligible trace amounts if any at all.

Also, if we look at the mechanism of this reaction, we’ll see the following resonance-stabilized intermediates (σ-complex) for each of our substitution positions. I purposefully didn’t show any resonance contributors with open shell oxygen as those would be negligibly minor contributors.
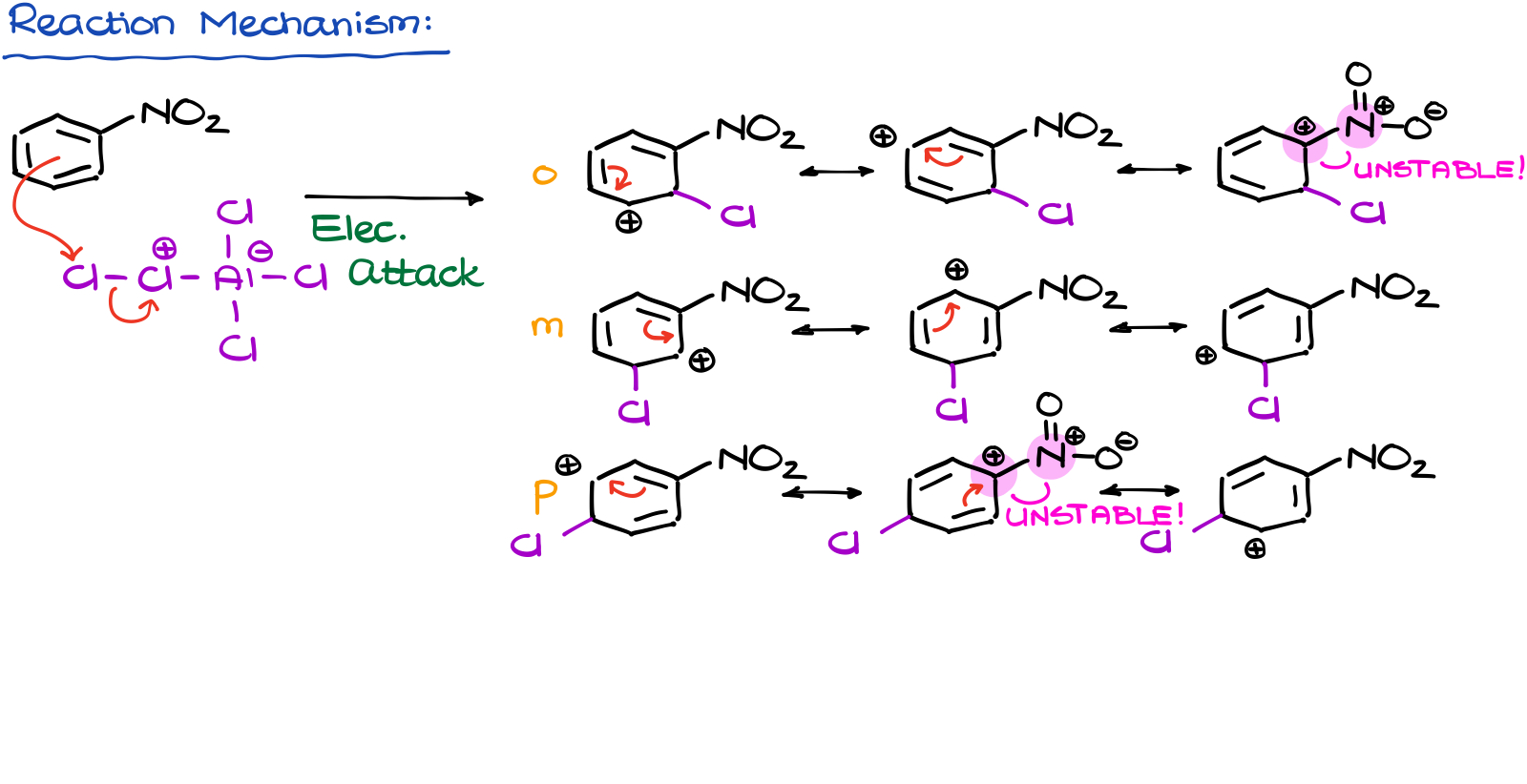
But even with those that I did show here, we see the situation where two positive charges are right next to teach other on the adjacent atoms. This is a highly unstable and thermodynamically unfavorable situation. Thus, the overall o/p-contributors are going to be less stable than the meta one. And since they are less stable, they’ll have a higher energy and will form slower.
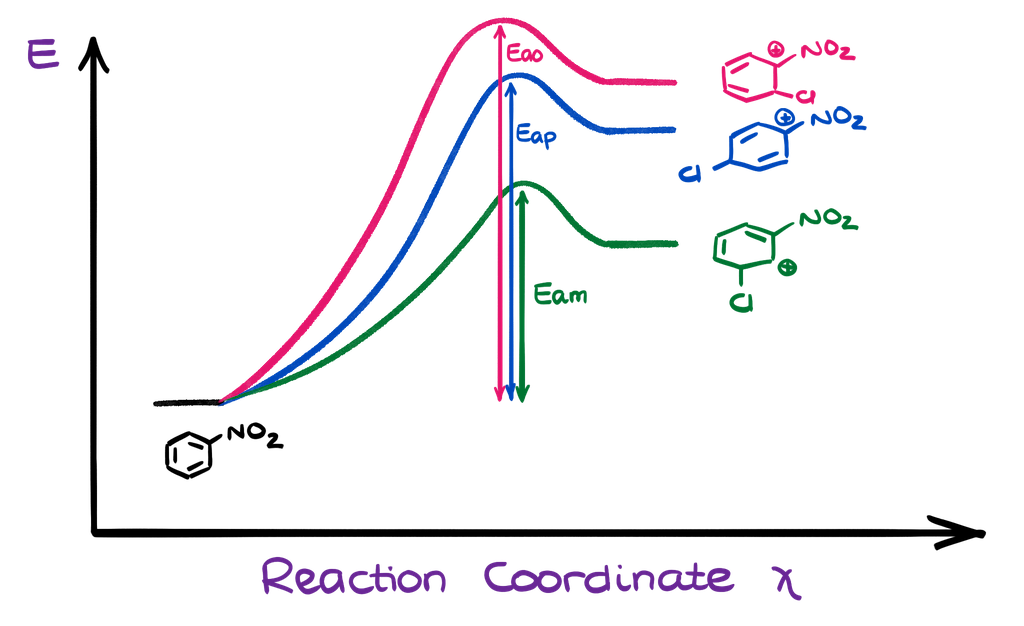
We can represent it with the energy diagram. From the energy diagram perspective, we can also see that the energy pathway leading to the formation of the meta intermediate has the lowest possible activation energy, so it will happen the fastest. So, the picture is pretty much opposite from what we’ve seen in the case of the activating and electron donating groups.
Now, just like for the donating and activating groups, we’re going to put the deactivating groups into three categories.
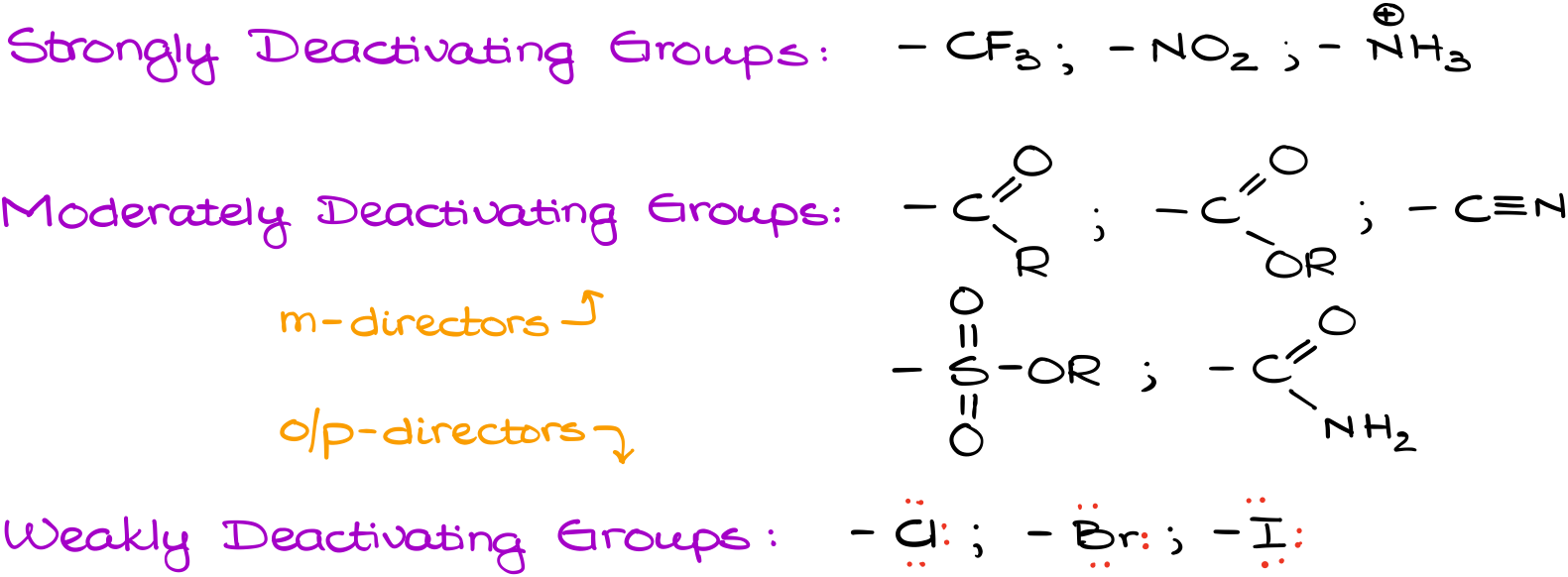
Strongly deactivating groups contain species with full positive charges like the nitro group or ammonium salts. The moderately deactivating groups are our carbonyls, carboxylic acid derivatives, and similar groups. And finally, the weakly deactivating groups are halogens.
And while all activating groups are o/p-director, only strong and moderately deactivating groups will be m-directors. The halogens, which are the weakly deactivating groups, still direct the substitution in the o/p-positions due to their weak resonance stabilization effect that they can provide for the carbocationic intermediate.
Activating and Deactivating Groups Reactivity Spectrum
So, we can put all types of groups we typically see in organic chemistry on the reactivity scale.
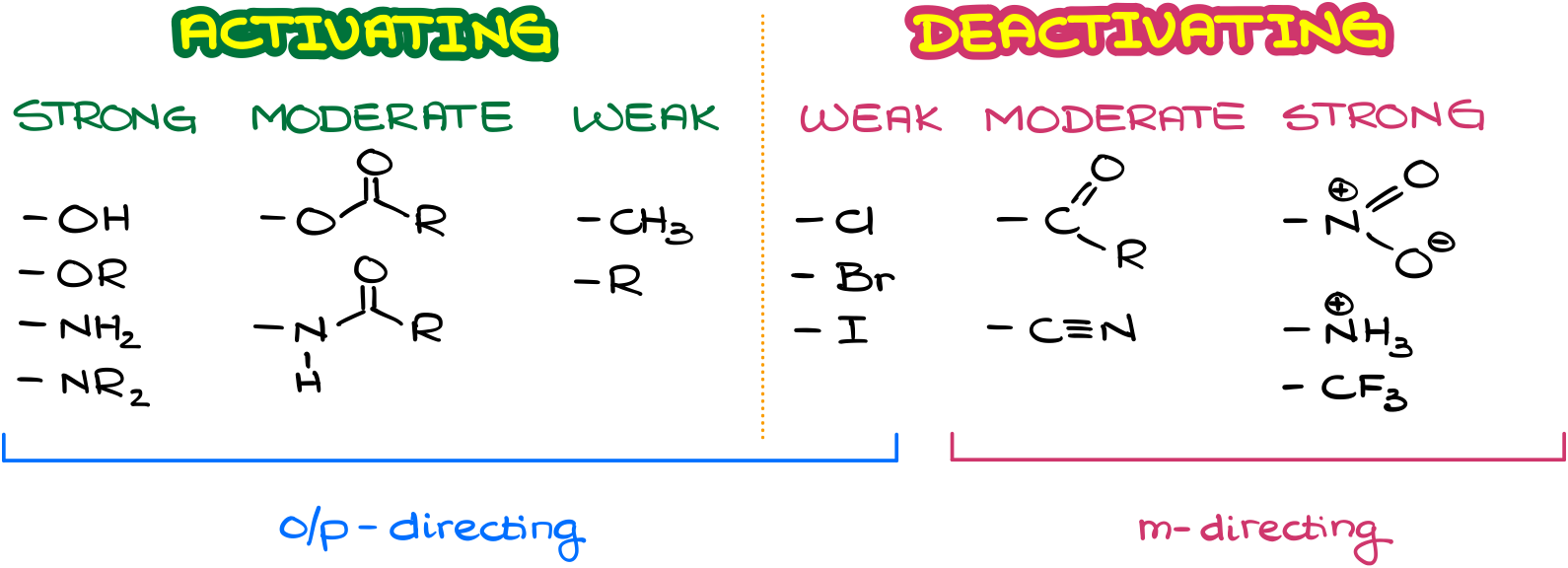
This is a rather useful little diagram, so you may wanna copy it down as you’ll need it in the future when you’ll be working on the multistep syntheses in which you’ll have several sequential electrophilic aromatic substitutions. It’s also a very common exam question when your instructor gives you an already substituted aromatic ring and asks you to predict the product of an electrophilic aromatic substitution using that molecule as a starting material.
Examples of Electrophilic Substitution Reactions with Different Directing Effects
For instance, here’s an example of the chlorobenzene reacting with nitrating mixture.
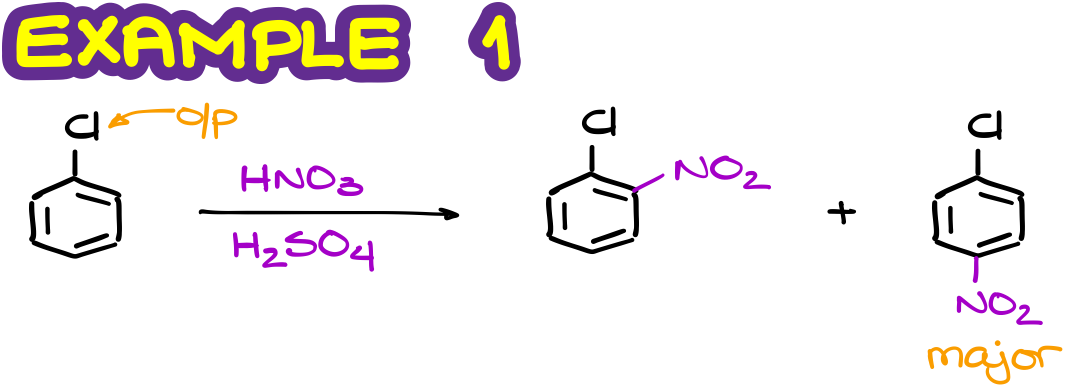
As chlorine is an o/p-director, we’ll do a substitution in the o/p-positions on our ring. We also remember that in the o/p-directed reactions, the major product is typically the p-isomer.
But if I were to do the halogenation of the nitrobenzene, my major product will be the meta-isomer. Also, since the nitro group is a strongly deactivating group, the second reaction will proceed significantly slower than the first one.
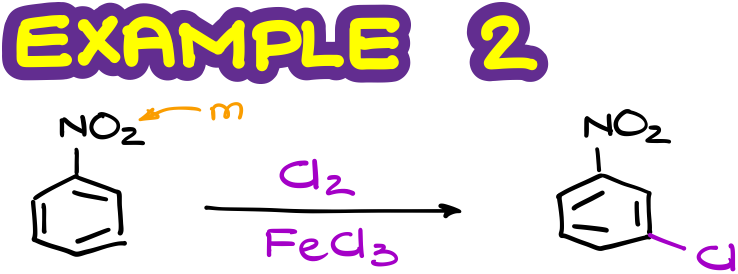
Here’s another example.
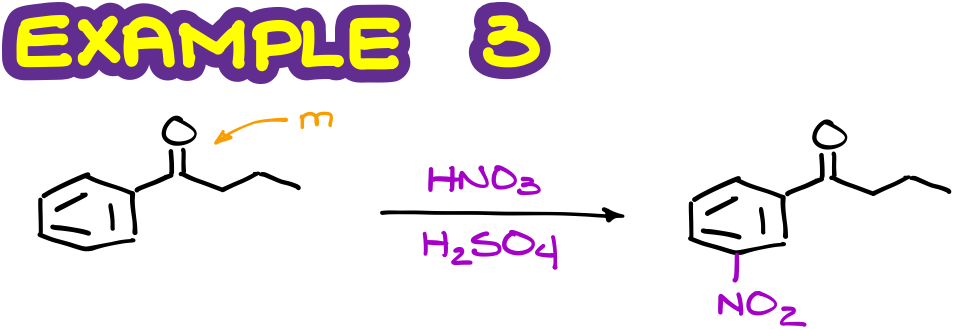
Since the ketone is a moderately deactivating group and a meta-director, we’ll expect the final product to be the meta-isomer.
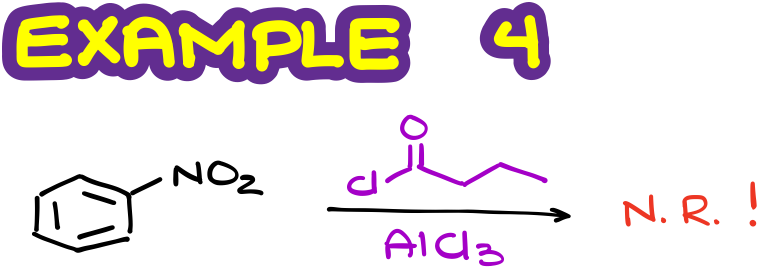
But if I switch my groups and attempt a Friedel-Crafts acylation here, we’ll get a big fat nope, as the Friedel-Crafts acylation doesn’t work for aromatic rings containing moderately or strongly deactivating groups.
That was a lot of information! But here’s a cool part: if you ever forget what sort of directing effect the group has, you can just draw the resonance structures for the molecule itself or the σ-complex and figure it out right there and then. Sometimes, finding the best position for the electrophilic aromatic substitution can be a bit of a challenge. But I promise you, the resonance will always help you find your way. That’s why I emphasize the resonance so much and keep going back to it again and again.