Another Tricky Epoxidation Exam Question
So here’s a pretty common exam question. You’re given a couple of molecules that both undergo the same reaction, likely giving a very similar product. And the question is: which reaction proceeds faster?
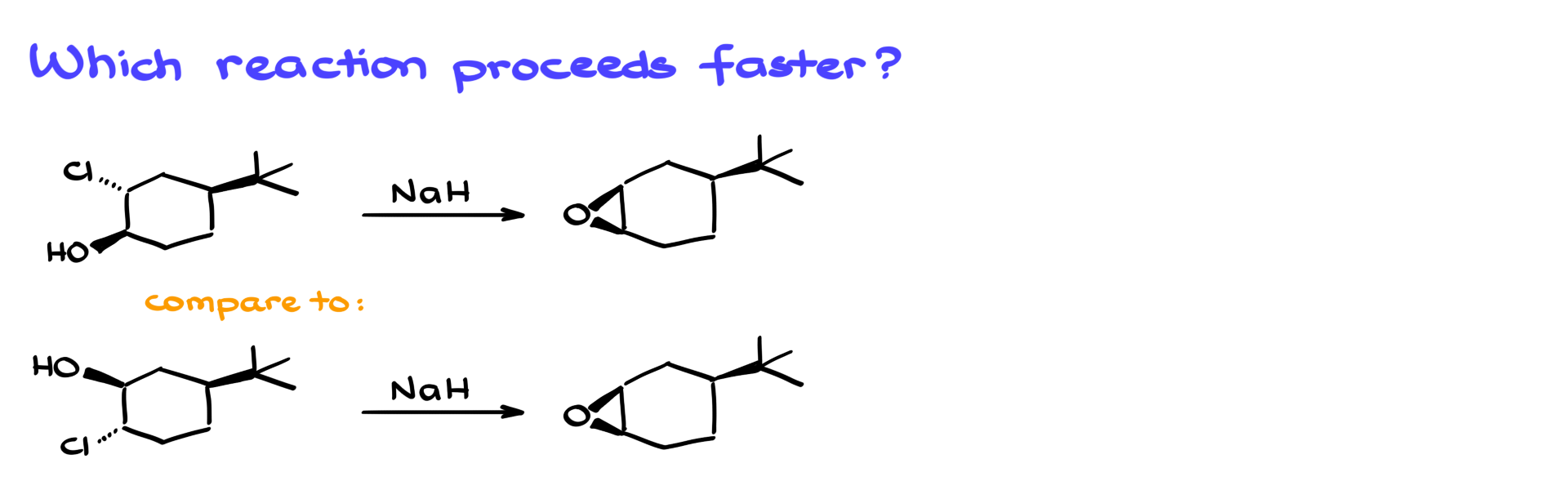
Well, first things first—it’s a good idea to figure out what exactly is going on here. We’re doing a reaction with sodium hydride, which is a very strong base. Generally speaking, we’re looking at a reaction where a halohydrin reacts with sodium hydride. The very first step is that the hydride ion, being such a good base, comes in and pulls off the proton from the alcohol group. That gives us an intermediate with an alkoxide.
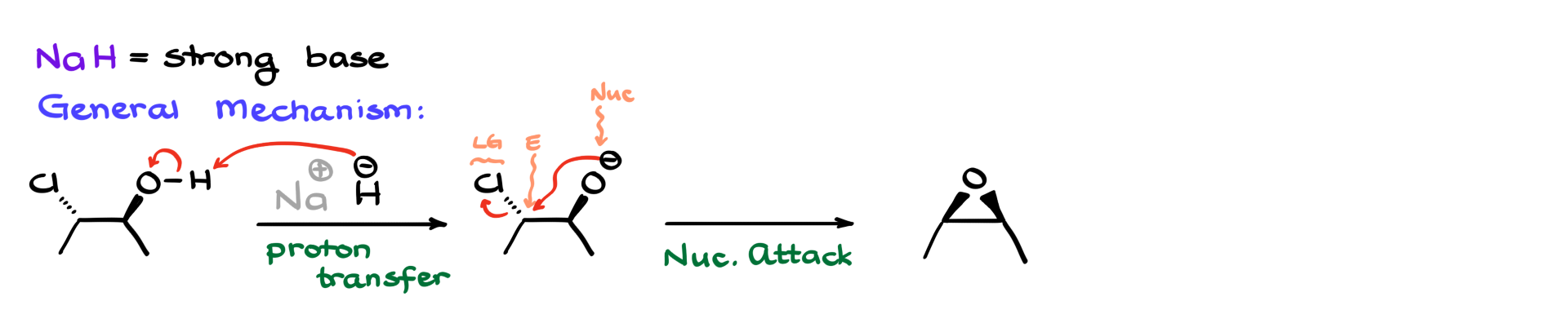
Now we have a nucleophile on one side and an electrophilic atom with a leaving group on the other—our chlorine in this case. So naturally, we’re going to see a reaction between the two: the oxygen attacks the carbon with the chlorine, kicks out the leaving group, and forms an epoxide as the final product. Pretty straightforward, right? And since both starting molecules lead to the same product, you might think the rate would be similar too.
But here’s a spoiler: the top reaction goes extremely fast, while the bottom one is painfully slow—so slow, in fact, that it barely even works. So what’s going on? How can one reaction be lightning fast and the other move at a glacial pace?
Let’s look at the chair conformations of our six-membered rings. For six-membered rings, chair conformations are key to understanding reactivity. I’ll draw the most stable chair conformation for both the top and bottom molecules. And since we’ve already removed that proton in the first step, I’ll erase the hydrogen and show the negative charge on the oxygen to reflect the alkoxide form in both cases.
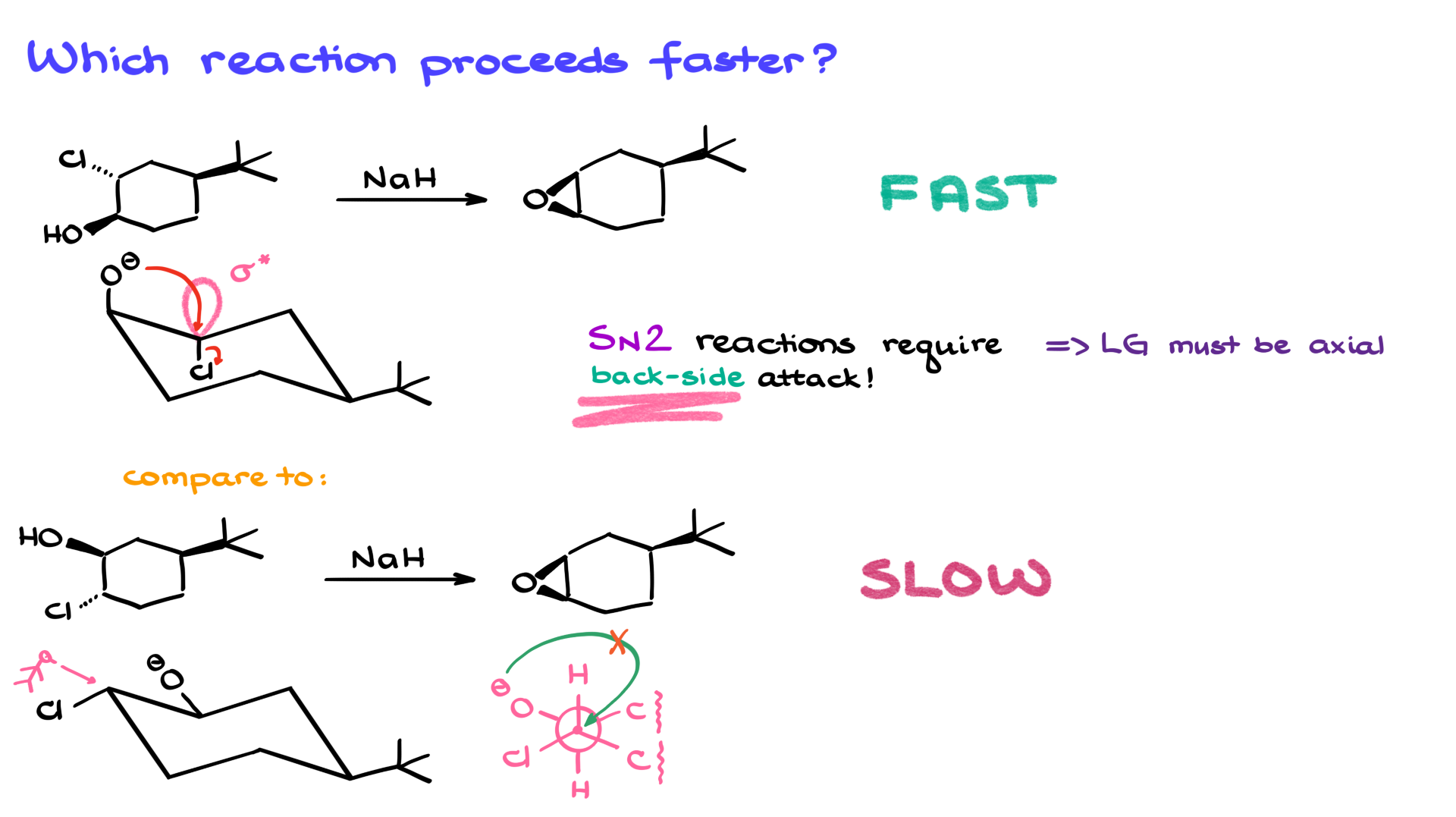
We’re dealing with an intramolecular SN2 reaction here. That means our oxygen is going to attack the electrophilic carbon from the opposite side, pushing out the leaving group. One of the absolute musts for SN2 reactions, especially in six-membered rings, is that the leaving group has to be axial. The reason? SN2 requires a backside attack, and that’s only possible if the anti-bonding orbital is aligned properly—180° from the nucleophile. If the leaving group isn’t axial, the anti-bonding orbital is tucked inside the ring and basically inaccessible.
In the top molecule, the most stable chair conformation already has everything lined up just right. The groups are positioned perfectly, the orbitals are aligned, and the reaction can proceed immediately and very quickly.
But the bottom molecule has a problem. If I draw a Newman projection along the carbon–carbon bond where the action is happening, here’s what I see: on the front carbon, there’s a hydrogen pointing up, a chlorine to the left, and a ring carbon to the right. On the back carbon, there’s another hydrogen pointing down, an oxygen to the left, and another ring carbon on the right. Now, for this reaction to happen, the oxygen would have to attack the carbon with the chlorine from the backside. But clearly, that alignment just isn’t there—it’s geometrically impossible.
So for the bottom molecule to react, it would first have to flip into a different, less stable chair conformation—one where the chlorine becomes axial. But here’s the kicker: because of the bulky tert-butyl group also present, that flip is basically impossible. The tert-butyl group strongly prefers the equatorial position, and forcing it axial to make the flip happen introduces way too much strain. So the bottom reaction doesn’t really go, while the top one takes off immediately.
The takeaway? Anytime you’re dealing with six-membered rings and a question involves reactivity, rate, or kinetics, you can bet chair conformations are playing a central role. So make sure you’ve got those down before your test—this is the kind of thing that shows up a lot.